Examining the Effect of Gelatin Concentration on Phantom Stiffness for Biopsy Training
- Pre-Collegiate Global Health Review
- Jul 2, 2023
- 9 min read
By Renee Ngai* and Nathan Hogaboom**
*Livingston High School, Livingston, New Jersey, United States
**Kessler Foundation, West Orange, New Jersey, United States
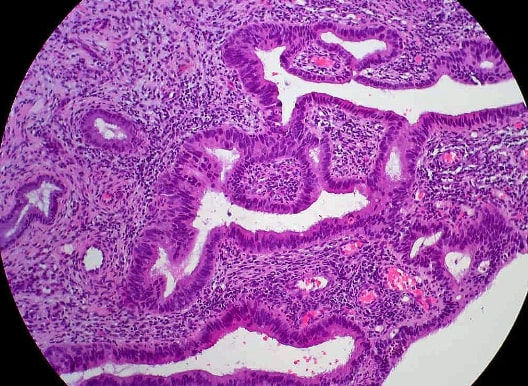
Abstract
Cancer is a global health battle, with a majority of cases being underdiagnosed in low-income countries. This treatment gap can be addressed with the development of an inexpensive cancer diagnoses tool. In particular, phantoms are tissue-mimicking materials that are used to train oncologists to perform ultrasound-guided biopsies. Phantoms need to reach a specific stiffness to mimic the right kind of tissue. Therefore, aim of this study is to examine the feasibility. The four phantoms of 18 g, 20 g, 22 g, and 24 g gelatin were refrigerated at 6 degrees Celsius. Phantom stiffness was measured with an ultrasound imaging device. An ANOVA test was conducted for phantom using inexpensive ultrasound phantoms by testing the stiffness of phantoms made from gelatin and psyllium husk. These materials are globally abundant and cost-effective, making them ideal for low-resource settings. A statistically significant difference was observed between the stiffness of four phantoms with different gelatin concentrations (F=3.49, p<.01). The results indicate that as gelatin concentration increases, the stiffness of the phantom increases, which meets the hypothesis. The 18 g phantom, which had a stiffness of 25 kPa, was found to be comparable to the stiffness of HeLa cell lines for cervical cancer. Such phantoms can offer low-cost biopsy training options for cancer diagnoses in underdeveloped countries where expensive manufactured phantoms are scarce.
Introduction
Cancer is a significant global health concern. According to the World Health Organization, this disease has been causing millions of deaths, especially due to a lack of cancer diagnosis training in underdeveloped countries (WHO, 2018). Specifically, 60% of all cancer deaths occur in underdeveloped regions (Negron, et. al. 2002). An inexpensive phantom, which is a tool for biopsy and ultrasound training, is therefore needed to reduce the number of cancer deaths in underdeveloped countries.
Ultrasound phantoms are tissue-mimicking materials that are used as a biopsy training aid and used in assessing ultrasound imaging devices (Bude and Adler 1995). There are two types of ultrasound phantoms. The first one mimics the acoustic properties (speed of sound, attenuation) of human tissue, and the second one mimics that of the appearance of the tissue. The ultrasound imaging phantom is used in testing ultrasound imaging devices. The other type of biopsy phantom is for biopsy training.
An ultrasound imaging phantom is a type of gelatin cube intended to mimic human tissue, and is expensive to produce since it requires constant stirring during cooling. On the contrary, the biopsy phantom is easy to produce and inexpensive, but they are transparent, so biopsy phantoms are not very useful in biopsy training since their specific stiffness differs from real human tissue, making biopsy artificially easy to perform (Bude and Adler 1995). In order to be useful in medical procedure training, phantoms have to achieve a specific stiffness and elasticity that is targeted towards their specific purpose.
In recent years, there is an increase in demand for ultrasound phantom models for biopsy and ultrasound imaging training, but ultrasound phantoms remain costly for radiology students (Richardson, et. al. 2015). Another limitation for ultrasound phantoms is that they are very expensive, and a single ultrasound phantom can only be used for a specific type of training, so different types of ultrasound phantoms with different levels of stiffness are needed. Purchasing numerous phantoms for ultrasound and biopsy training can be very expensive, especially for healthcare institutions in underdeveloped countries. Developing an inexpensive ultrasound phantom would therefore provide accessible biopsy training around the globe.
Hypothesis
Since underdiagnosis of cancer cases is a global health concern, developing a novel formula for making inexpensive ultrasound phantoms with the desired stiffness is needed. This leads to the research question: what effect does the mass of gelatin used in creating an ultrasound imaging phantom have on its properties (stiffness, physical appearance, and mass)? This study hypothesized that the increase in gelatin concentration will result in an increase in phantom stiffness.
Methods
Materials needed include boiling water, Knox gelatin, psyllium husk, and a plastic container (Figure 1). Three boxes of Knox gelatin and a carton of sugar-free psyllium were obtained from a grocery store. Boiling water was obtained from the sink, and the plastic container was obtained from an arts and crafts store.
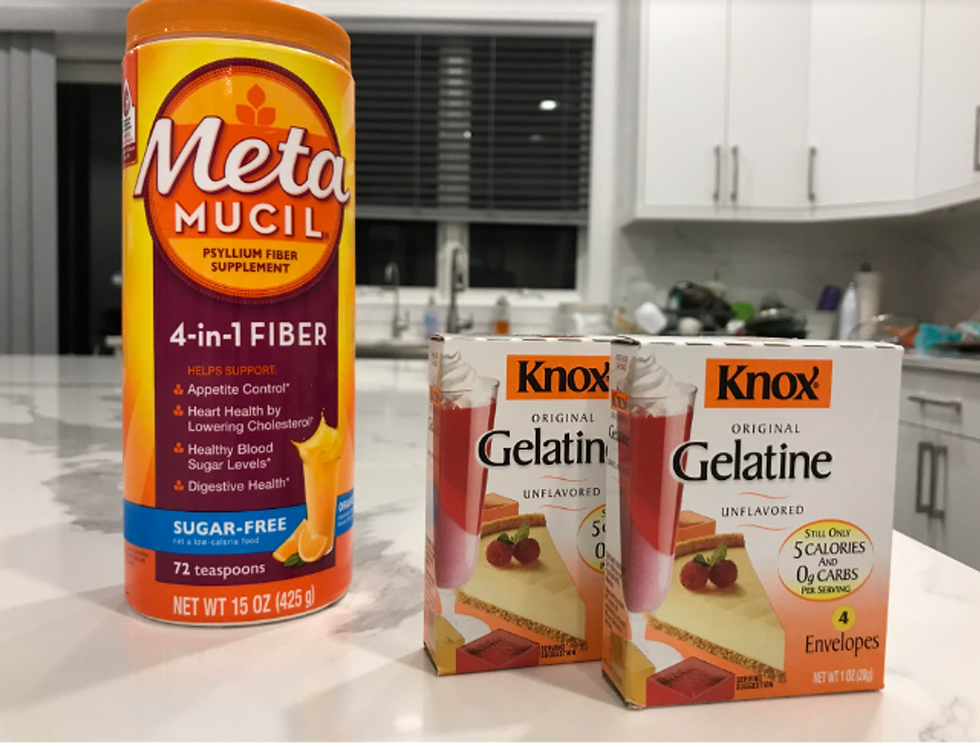
Figure 1: An image of the ingredients needed to make a low-cost ultrasound phantom. Main ingredients include psyllium husk, gelatin, water, and a container.
To make the ultrasound phantom, four bowls are lined up: from 24, 22, 20, and 18 grams of gelatin, respectively, from left to right (Figure 2) 1 cup of boiling water and 10 grams of psyllium husk is then added into each of the white bowls. The mixtures are stirred until the psyllium husk and gelatin are thoroughly dissolved, and the solution is poured into a cubic container of 6 cm wide, 8 cm long, and 7 cm tall. The mixture is stored in the refrigerator for 1 hour at 6 degrees Celsius until the layer is firm.
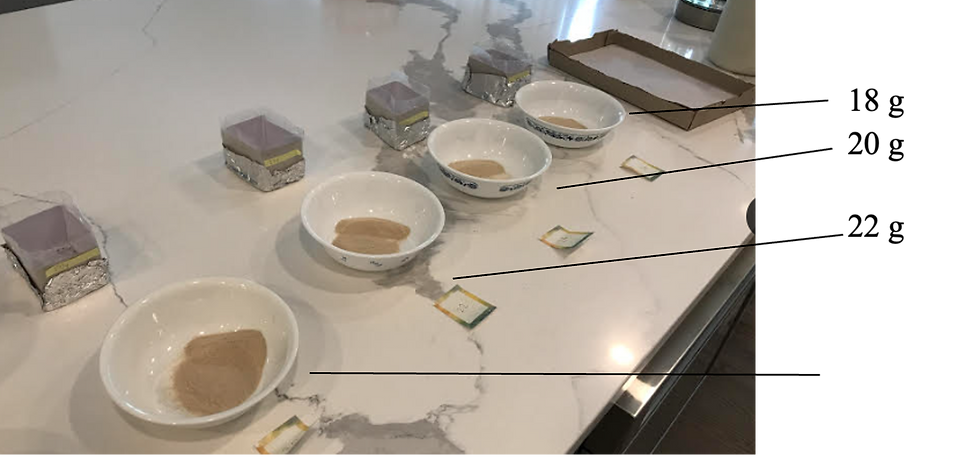
Figure 2: An image of the experimental set up. The white bowls are filled with 10 g of psyllium husk and 18, 20, 22, and 24 g of gelatin, respectively. Boiling water is added to the white bowl, and the stirred mixture is put into plastic containers behind the bowls.
After the phantom was made, images were collected using an Aixplorer Mach 30 ultrasound machine. The phantom is placed under a Superlinear 5-18 MHz ultrasound imaging probe, and an image of the phantom was obtained. Ultrasound images will be taken for the ultrasound phantom, as well as the ordinary appearance of the phantom under natural light. After the ultrasound phantom is made, the data collection will be done at Kessler Foundation. For the dependent variable, the elasticity of the ultrasound imaging phantom, collected with an ultrasound imaging using ultrasound shear wave elastography.
Since the independent variable is categorical, and the dependent variable is quantitative, an ANOVA test will be conducted to look at whether there is a difference in the average stiffness in 4 categorical groups: 18 grams of gelatin, 20 grams of gelatin, 22 grams of gelatin, and 24 grams of gelatin. A T-test will also be carried out to examine the statistical difference in the average mass of the phantoms for different gelatin concentrations. Lastly, an ANOVA test will be conducted to look at whether there is a difference in the average mass in phantoms with different gelatin concentrations. Descriptive statistics such as the mean, the median, the mode, and the standard deviation will also be calculated for the stiffness, viscosity, and elasticity of the ultrasound phantoms.
Results
Physical Appearance of Ultrasound Imaging Phantoms
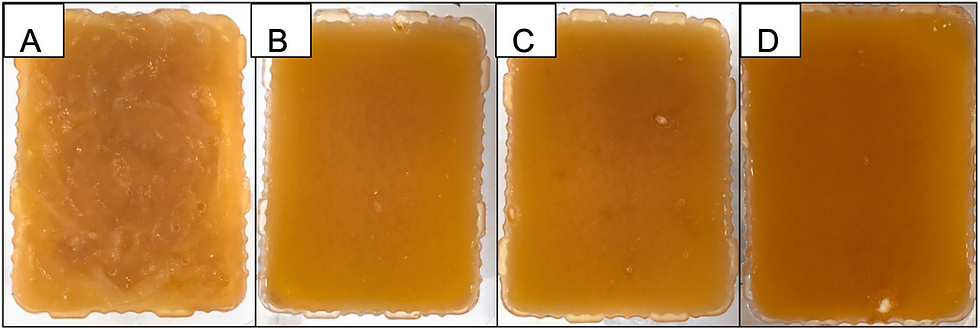
Figure 3: Physical appearance of the ultrasound phantoms in accordance to different gelatin concentrations. Figure 3a is 18 g of gelatin, 3b is 20 g of gelatin, 3c is 22 g of gelatin, and 3d is 24 g of gelatin.
The surface appearance of the four phantoms shows that as the gelatin concentration increases, the surface appearance is smoother and has less impurities on the surface. For example, Figure 3a has the least gelatin content of 18 grams, so the surface image appeared to be rough and coarse. Figure 3d, on the other hand, is the phantom with the most gelatin, and the surface of the 24 g gelatin phantom is the smoothest, with the least impurities. The results align with the direct relationship between gelatin content and surface smoothness, since as the gelatin content increases, its surface smoothness increases. A potential explanation for this may be because as the gelatin cools, new bonds form between the amino acids in the protein, and more gelatin will lead to more bonds forming, thus leading to a smoother surface.
Ultrasound Elastography Images of Phantoms
The graph shows that the Figure 4 above shows the surface appearance of the ultrasound phantoms in accordance to different gelatin concentrations. Figure 8 represents the phantoms with 18 g gelatin, 20 g gelatin, 22 g gelatin, and 24 g gelatin, from a to d, respectively. White impurities are evenly spreaded within all of the phantoms, which represents the dissolved psyllium husk in the phantom mixture. The ultrasound images of all the phantoms shows that as the gelatin concentration increases, the stiffness of the phantom increases. For instance, figure 4a has the least gelatin content of 18 grams, so the ultrasound image is blue, meaning the stiffness is about 26 Kilopascals. Figure 4d, on the other hand, is the phantom with the most gelatin, and the color of the 24 g gelatin phantom is red, meaning the stiffness is about 52 Kilopascals. The results agree with the direct relationship between gelatin content and stiffness, since as the gelatin content increases, its stiffness increases. Potential explanations may be because as the gelatin cools, new bonds form between the amino acids in the protein, and more gelatin will lead to more bonds forming, thus leading to a stiffer phantom.
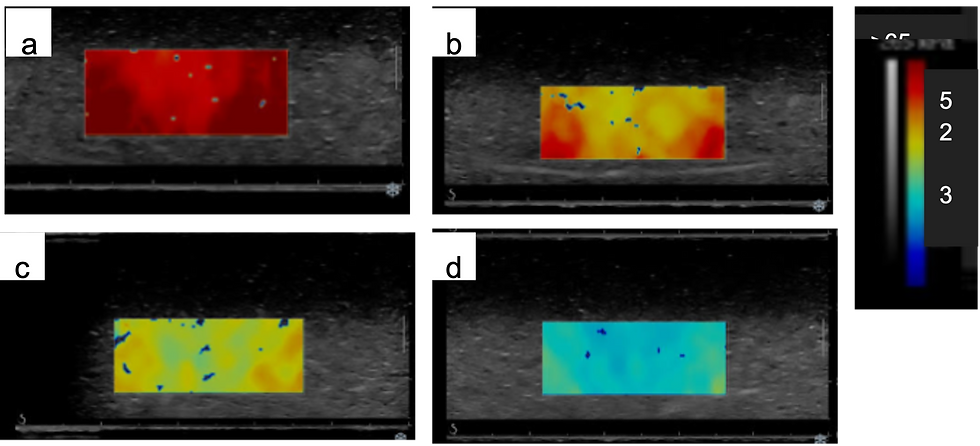
Figure 4: An ultrasound image of the four phantoms. Figure 4a is an image of the 24 g gelatin phantom, figure 4b is a 20 g gelatin phantom, figure 4c is a 22 g gelatin phantom, and figure 4d is a 18 g gelatin phantom.
ANOVA Test for Stiffness of Gelatin Phantoms
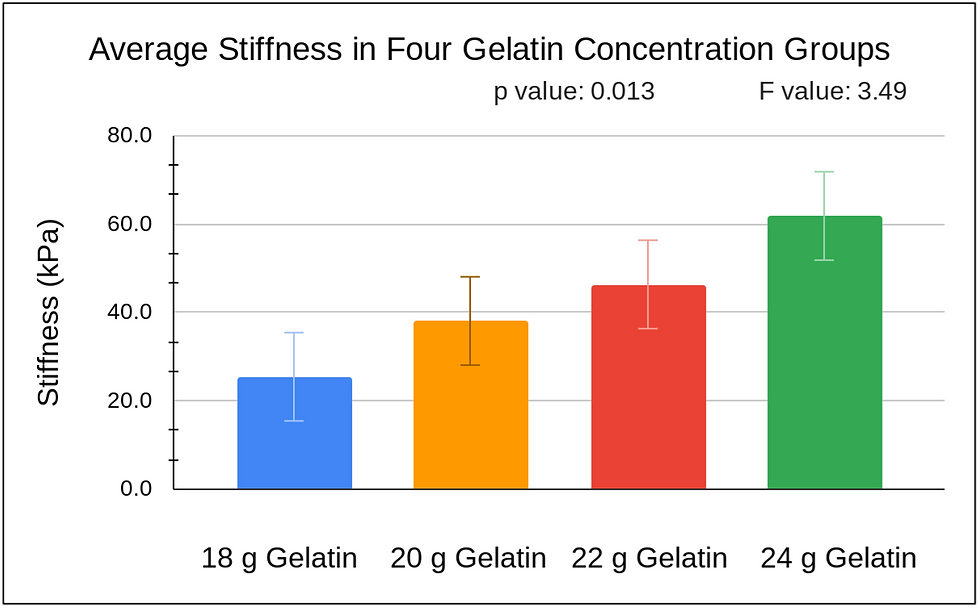
Figure 5: A bar graph of the average stiffness in four groups with different gelatin content. Stiffness increases as gelatin content increases.
Stiffness increases when the gelatin content increases. For an ultrasound phantom with 24 grams of gelatin, the stiffness of the ultrasound phantoms is highest at 61.9 Kilopascals. On the other hand, with only 18 grams of gelatin, the stiffness is lowest at 25 Kilopascals (Figure 5). An ANOVA test was conducted, and the p-value is 0.0013 and the F value is 3.49, which may indicate that there is a statistically significant difference between the stiffness of four groups of phantoms with different gelatin concentrations. Potential explanations may be because the increase in gelatin concentration increases the bonds formed between the amino acids in the phantom, which increases the rigidity of the phantom. The results are relevant to the research question because it indicates that an increase in gelatin content is correlated with an increase in phantom stiffness.
T-Test for Stiffness of Gelatin Phantoms
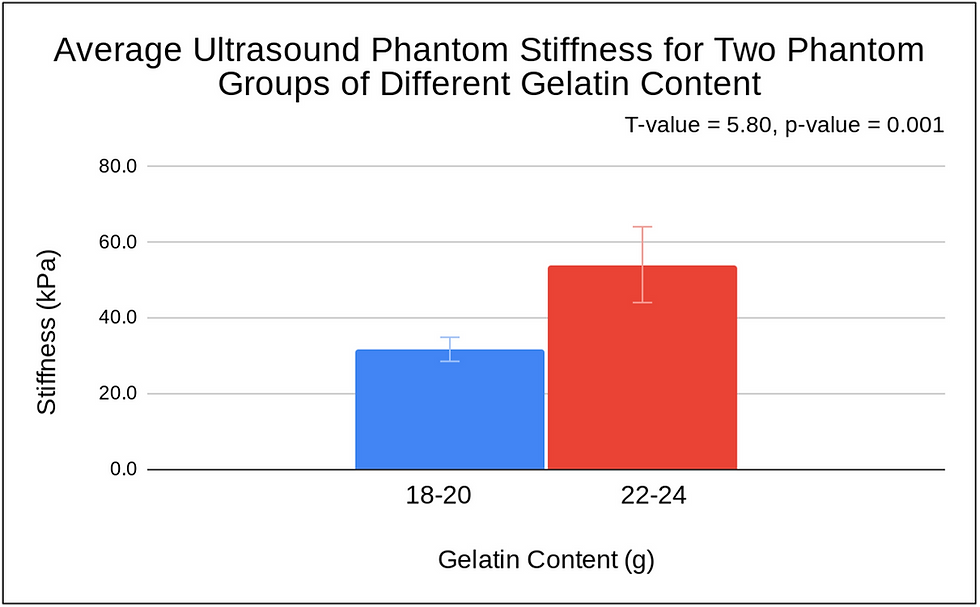
Figure 6: A bar graph of the average phantom stiffness for two groups of different gelatin content. As gelatin concentration in a phantom increases, the stiffness of the phantom increases.
Stiffness increases when the gelatin content increases. For an ultrasound phantom with 22-24 grams of gelatin, the stiffness of the ultrasound phantoms is highest at 61.9 Kilopascals. On the other hand, with only 18-20 grams of gelatin, the stiffness is lowest at 25 Kilopascals (Figure 6). A T-test was conducted, and the p-value is 0.001 and the F value is 5.8, which may indicate that there is a statistically significant difference between the stiffness of two groups of phantoms with different gelatin concentrations. Potential explanations may be because the increase in gelatin concentration increases the bonds formed between the amino acids in the phantom, which increases the rigidity of the phantom. The results are relevant to the research question because it indicates that an increase in gelatin content is correlated with an increase in phantom stiffness.
Conclusions
An inexpensive phantom, which is a tool for biopsy and ultrasound training, can be utilized to reduce the number of cancer deaths in underdeveloped countries. Our results indicate that as gelatin concentration increases, the surface smoothness, stiffness, and mass of the gelatin phantom increases. The proposed question of finding the relationship between gelatin and its effect on phantom stiffness was answered since an ANOVA test was conducted for phantom stiffness, and the p-value is 0.0013 and the F value is 3.49, which may indicate that there is a statistically significant difference between the stiffness of four groups of phantoms with different gelatin concentrations. The hypothesis that an increase in gelatin concentration is related to an increase in phantom stiffness was met.
There are several implications of this ultrasound phantom experiment. First, the 18 gram gelatin phantom has a stiffness of 25 kPa, which is equivalent to the average stiffness of HeLa cell lines for cervical cancer (Kwon, et. al. 2020). Therefore, the 18 gram ultrasound phantom can be used for cervical cancer ultrasound-guided biopsy training. Also, the 22 gram gelatin phantom can be used in lung cancer biopsy training, since the 22 gram gelatin phantom has a stiffness of 38 kPa, which is the stiffness of the H1299 cell line, a lung cancer cell line derived from the lymph node that is widely used in research (Kwon, et. al. 2020). These two phantoms can offer low-cost biopsy training options for cancer diagnoses in underdeveloped countries where expensive manufactured phantoms are scarce. To make phantoms with the desired stiffness, the best fit equation found in this experiment (phantom stiffness = 5.87 x gelatin content - 80.4) can be followed.
The implications stated above would lead to more training in cancer diagnosis in low-income countries and combat the global health concern of rising cancer cases globally. The new phantom would also reduce the gap in cancer care globally.
One source of error includes pressing too hard on the phantom when taking ultrasound images of the phantom, leading to a slightly higher stiffness for the 20 g gelatin phantom. Pressing too hard on the phantom will result in a higher stiffness than normal, since pressing on the phantom condenses the phantom. Another source of error includes spilling a pinch of gelatin on the table when pouring the gelatin into the mixing bowl, which led to a slightly lower stiffness for the 22 g gelatin phantom than the predicted amount. Limitations include a small sample size for the stiffness, since the stiffness of the phantoms were only measured for four times. For future studies, a larger sample size can be included to derive a more accurate relationship between phantom stiffness and gelatin concentration.
References
Comments